Current State of Stem Cell Treatments for Cerebral Palsy: A Guide for Patients, Families, and Service Providers
Stephanie Beldick and Dr. Michael G. Fehlings , 2017
Please note that terms in bold are defined in the glossary, which can be found at the bottom of the page.
Stem Cells and Cerebral Palsy
Cerebral palsy (CP) is an umbrella term used to describe a disorder that results from perinatal brain injury. CP can be caused by a multitude of factors, including premature birth, infection in the uterus, lack of nutritional support during development, lack of oxygen at the time of birth, and genetic aberrations. While the cause of CP is multifactorial, the injury consistently leads to various neuro-motor deficits, often accompanied by other symptoms, such as visual and cognitive impairments.
When an insult occurs to the brain during the sensitive perinatal period, the resident brain cells are unable to promote proper growth and development of the brain. Neurons and oligodendrocytes die and/or fail to mature, and the white matter tracts that connect various brain regions become damaged. Importantly, the corticospinal tract (CST), which connects the motor regions of the brain to the spinal cord and helps control movement, is often damaged. Without functional CST connections, motor deficits ensue.
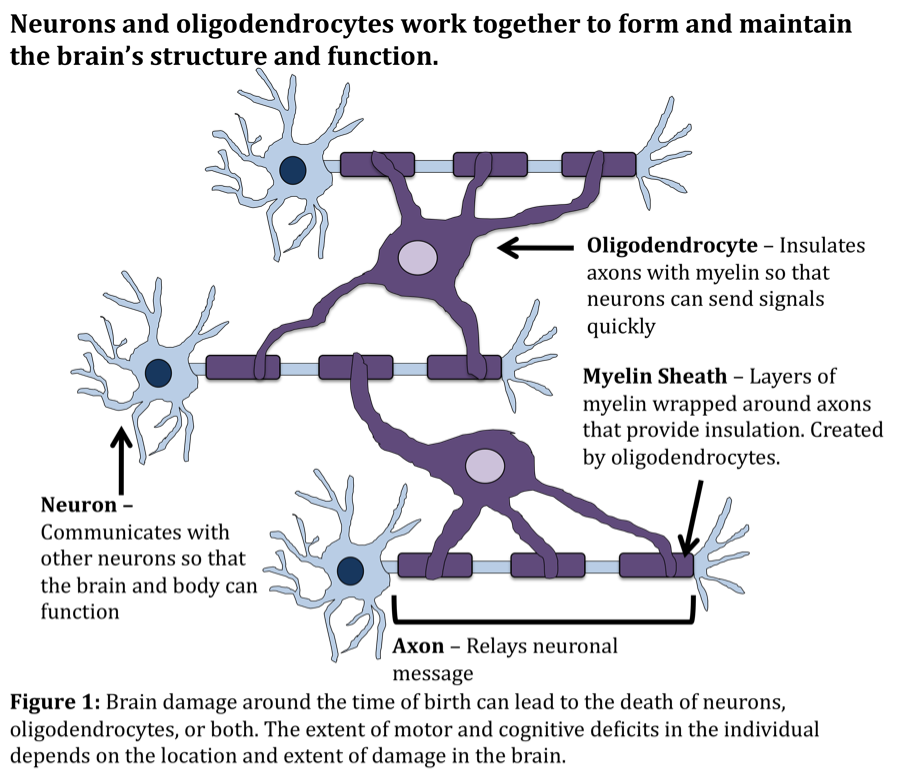
Stem cell transplantation is a regenerative therapy that has the potential to replace the damaged and non-functional cells in the brains of CP patients, as well as to provide support to the remaining neurons and oligodendrocytes. There are many kinds of stem cells, each with different and unique characteristics.
As research has advanced, we have discovered that stem cells can be induced to become more specialized cell types, and when transplanted into the body, they can provide support to a damaged environment. In addition, we can modify these stem cells to express certain factors that can enhance this ability. We can also modify the cells to express scar-degrading factors that can help to reduce scarring in the brain and promote recovery.
There is a plethora of research in the field of stem cell transplantation for CP, and this will be discussed below in the context of both preclinical animal research and clinical trials.
What are Stem Cells?
There are two characteristics that make stem cells unique from other cells in the body. First, they have the ability to divide and make copies of themselves over extended periods of time. Second, they can differentiate into more specified functional cell types. This means they can transform into specialized cell types of the body such as heart, lung, or brain cells. The two characteristics of long-term cell division and differentiation capacity have some very important implications for regenerative therapeutic strategies. The ability of stem cells to create copies of themselves over long periods means that their supply is theoretically limitless; under optimal conditions, a stem cell can be grown in a culture dish and end up forming colonies of stem cells. After generating a large pool of stem cells, they can be directed to transform into more specialized cell types, assuming the scientist knows the correct factors that will lead to the desired change. Below, we will discuss some of the most important stem cell types relevant to the treatment of CP, as well as their pros and cons for use in research and the clinic.
Embryonic stem cells (ESCs) are found in the developing embryo. These cells are known as pluripotent, meaning they have the potential to differentiate into all the cell types found in the human body. ESCs have been studied extensively in order to understand the molecular pathways that regulate their “stem cell-ness”, along with those pathways that guide differentiation to more specialized cell types (for example, how does one ESC become a neuron and another a skin cell?). ESCs have unique potential for repairing damaged tissue because of their versatile differentiation capacity. This means that ESCs could be used to treat a vast number of disorders. However, scientists are still working to piece together the correct biological codes that can fully differentiate an ESC into the desired cell type. A consequence of not yet understanding the full molecular picture is that ESC-derived cells can sometimes revert back into pluripotent cells and form tumours. Another drawback is that the derivation of ESCs requires the use of cells from the embryo, thus raising moral and ethical dilemmas.
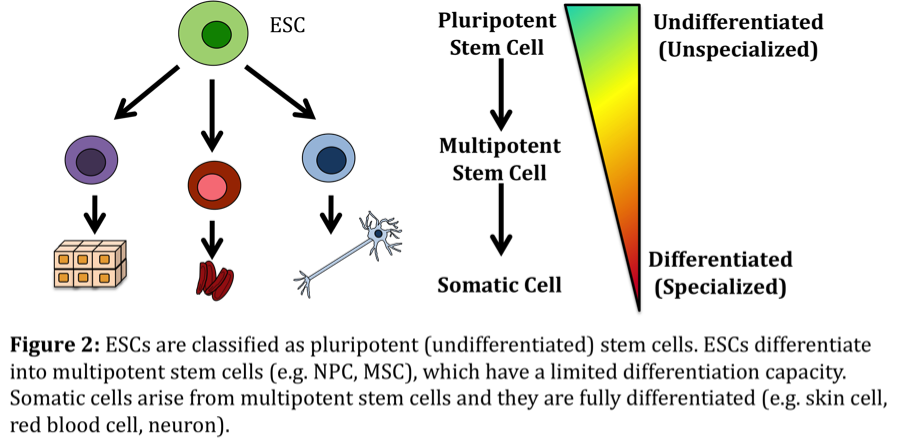
In 2008, Professor Shinya Yamanaka won the Nobel Prize for discovering four biological factors that could be used to turn any somatic (fully differentiated) cell into a pluripotent stem cell. These new, ESC-like cells were termed induced pluripotent stem cells (iPSCs). Following this discovery, there was a great excitement in the scientific community. Being able to create pluripotent stem cells essentially eliminates the need for embryonic tissue in order to obtain pluripotent stem cells. This was not the end of the excitement though; Normally, if a patient is to receive a stem cell transplantation, a donor for these cells is required (allogeneic transplantation). iPSCs, however, can be derived from the patient’s own somatic cells. First, a biopsy of somatic cells (e.g. skin cells) would be taken; the cells would then be induced into iPSCs in a culture dish and allowed to divide. Following division, the iPSCs could be differentiated into any cell type and then placed back into the patient (autologous transplantation) Not needing a donor to acquire the cells significantly reduces the risk of rejection following transplantation into the patient. Despite the enthusiasm surrounding these cells, iPSC-derived cells still present a risk of tumour formation, similar to ESCs. Further research is needed to better understand the molecular pathways underlying “stem cell-ness” and differentiation processes so that the safety and efficacy of these cells can be optimized for regenerative therapies. Interestingly, in recent years, new technology has allowed for the investigation of directly reprogrammed cells. In this case, somatic cells can be directly transformed into the desired cell type, essentially by-passing the pluripotent stage that is required when using iPSC technology. One benefit of these directly reprogrammed cells is the reduced risk for tumour formation; however, further research is needed in order to fully understand how we can generate these cells in a safe and efficient way.
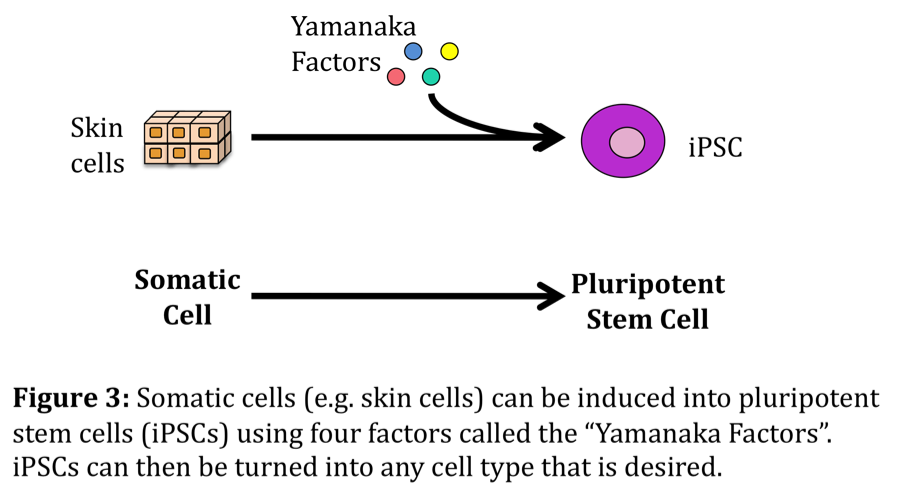
While ESCs and iPSCs are excellent sources for versatile cells, they are not convenient for transplantation on their own (due to tumour formation), and so they are nearly always induced to differentiate into more specialized cell types before transplantation.
Neural precursor cells (NPCs) are stem cells that are found in humans within the central nervous system (CNS). These stem cells are more differentiated than pluripotent stem cells, in that they can only differentiate into the cells that are found in the CNS. Accordingly, they are called multipotent stem cells. NPCs can be taken from a donor or the patient, grown in a culture dish and then transplanted into the patient. NPCs have a unique benefit over other cell types to treat CP; their ability to differentiate into brain cells provides them with the greatest potential to appropriately integrate into the injured brain and replace the damaged and nonfunctional cells. However, the drawback to using NPCs is that there is a limited supply of these cells, and while they can be grown in culture, the method of retrieval from the brain or spinal cord is very invasive. In addition, these cells are incredibly complex and there is limited research on their use for treating CP. Conversely, some benefits include: no ethical dilemmas with their derivation, they can be taken from the patient so the risk of rejection is minimized upon transplantation, and the time required to culture the cells is shorter than for pluripotent stem cells, since they are already in the desired format.
Mesenchymal stromal cells (MSCs) are another kind of stem cell that have been used in research surrounding CP. These cells have two main origins, either from the bone marrow (BMMSCs) or from the umbilical cord blood (UC-MSCs). Like NPCs, MSCs are multipotent, and they have the potential to differentiate into various cell types that play structural and immune roles in the body. MSCs have been used in research and the clinic for decades. Their benefits lie in their easy accessibility and their immune-modulation properties. However, MSCs lack the full potential to fully differentiate into functional cells of the CNS, and for this reason, they are unlikely to be the major focus of future multipotent stem cell research for CP.
Sources of Stem Cells for Transplantation
When discussing transplantation strategies, all stem cells, regardless of their type, can either be classified as allogeneic or autologous. Allogeneic cells are those taken from a donor and given to a patient, whereas autologous cells are derived from the patient. Allogeneic stem cells have benefits in that large stem cell banks can be created that can freeze and store stem cell donations for extended periods of time. When needed, the cells can be thawed and given to the patient in a short amount of time. A downside to allogeneic cells, however, is that there is a high risk of immune rejection upon transplantation, since the cells come from a donor. Conversely, autologous stem cells pose minimal risk for immune rejection, since they come from the patient. However, the process for extracting the cells, and culturing them can be lengthy, and if cells are needed within a short time frame, this method is inconvenient.
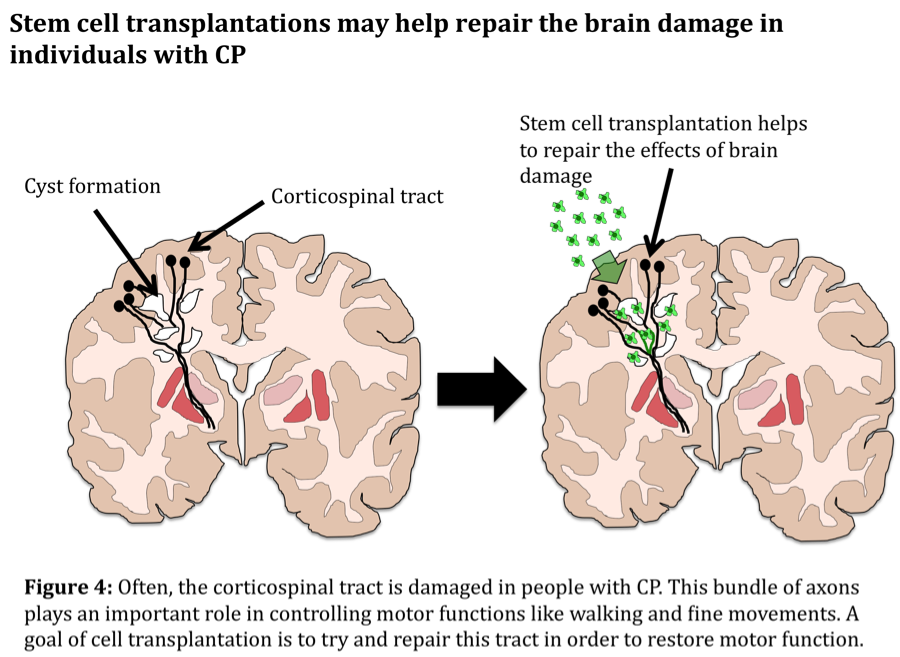
Preclinical Stem Cell Research
CP researchers have been utilizing various cell types, including ESC- and iPSC-derived NPCs, MSCs, as well as multipotent NPCs found in the brain. Results have been promising in that stem cell transplantation has resulted in modest functional improvements and positive anatomical changes within the brain.
As a part of the Kids Brain Health Network preclinical research team, Dr. Michael Fehlings and his laboratory (Krembil Research Institute, University Health Network and University of Toronto) are currently investigating NPCs and human iPSC-derived NPCs for the treatment of CP. The team is developing reproducible animal models of CP and injecting these stem cells into the brain. Importantly, the focus of this research is to use stem cells as a treatment in a chronic injury context. This means that we are designing stem cell therapies that will be efficacious long after the brain injury has occurred.
In preclinical models a few of the hurdles that need to be overcome are: improving cell survival in the brain following transplantation, reducing the risk of tumour formation (in the context of pluripotent-derived cells), and understanding how we can force our transplanted cells to integrate better into the existing neuronal pathways.
Despite these challenges, the knowledge gleaned from how stem cells function and incorporate into the damaged CNS is incredibly useful. This information has helped researchers to advance their strategies for cell transplantation, as well as to better understand the roles of endogenous NPCs (stem cells found within the brain) following brain injury.
Stem Cell Therapy Clinical Trials
Currently there are 12 clinical trials around the world using stem cells to treat CP. Four of these are recruiting, one is active but not recruiting, and seven have been completed. Many of these trials are making use of bone marrow/blood-derived mononuclear cells (a combination of cells originally found in the bone marrow that contain hematopoietic stem cells (stem cells that give rise to red and white blood cells, also termed HSCs) and BM-MSCs). Others are using purified HSCs, or umbilical cord blood containing UC-MSCs and HSCs. Many of these trials have passed the initial phases of clinical trials that only seek to evaluate the correct dosage and safety of the intervention. These trials are actually beginning to test the efficacy of these stem cells as treatments for CP.
To date, the findings of only one study have been published. This study had three groups of child participants: Those receiving conventional rehabilitation therapy only, those receiving a drug called erythropoietin (shown to have promise in treating CP) along with conventional rehabilitation therapy, or those receiving umbilical cord blood (containing stem cells) + erythropoietin + conventional rehabilitation therapy. The study found that the group who received stem cells showed greater improvements on cognitive and motor assessments when compared to the other treatment arms.
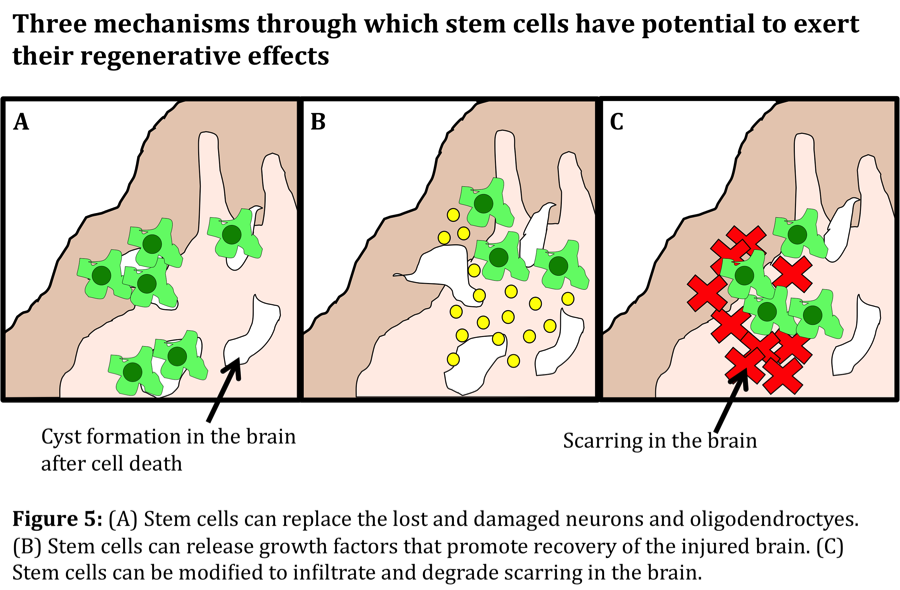
While the growth in the number of clinical trials since the last edition of this manuscript in 2011 (4 vs. the current 12) is exciting and holds promise for the future of stem cell therapies for CP, there is still a long way to go. None of the clinical trials to date have used NPCs or iPSC-sourced cells, which, as discussed above, have unique advantages. NPCs have the greatest potential to integrate into the damaged brain, and being able to use iPSC-derived cells will heavily reduce the need for stem cell donors, thereby minimizing the risk of rejection upon transplantation into the patient. iPSCs also hold the potential to be differentiated into any cell type. This versatility will be quite useful as more research is conducted on different cell types for the treatment of CP. However, learning how to fully control both NPCs and iPSCs and maximize their potential is an ongoing area of research that requires a lot of time and money. Researchers have been working hard though, and with the help of extensive collaborations, funding, and dedication, the world’s first iPSC clinical trial (RIKEN trial for age-related macular degeneration), put on hold in early 2015, has resumed.
Manufacturing and Regulatory Issues
Despite the progress seen with clinical trials in recent years, there are some hurdles that need to be overcome in order for stem cells to become a widely accessible treatment. One of the major issues currently facing the stem cell community is the problem of “scale up”. In the laboratory, stem cells are cultured in Petri dishes. It is feasible to use this approach to treat one or two patients, but as stem cell therapies come closer to the clinic there is an increasing need to develop strategies to manufacture cells on a large scale. Tools called bioreactors are a promising approach to solve this problem, however, with new large-scale production strategies, the protocols for cell production will need to be re-evaluated and the efficiencies of cell production will change. More research is needed in order to better understand how we can produce our desired stem cells in mass quantities.
The other problem is that regulatory agencies, such as the FDA and Health Canada are having difficulty developing standardized guidelines for the production and use of stem cells. Not only will individual regulations be required for each unique cell type, but also cells are a completely different biological therapeutic when compared to conventional drugs. Cells are living entities and their effects on the human body will not be as well defined. There is an urgent need to better establish these guidelines, as well as to standardize the manufacturing process in order to reduce variability among the cells during production. At the basic science level, some of these challenges will be overcome by improving our understanding of the mechanisms underlying how the cells work. Basic scientists, clinicians, industry partners, regulatory agencies, and patient advocates all need to work together to help stem cell therapies move forward.
Stem Cell Tourism
While the North American medical community is working to better establish stem cell therapies for CP, it is important to be cautious of unregulated overseas stem cell clinics. The act of going to these unregulated clinics is called “stem cell tourism”. While it may seem tempting to do so, and the testimonials on these clinics’ websites look promising, take heed; Stem cell transplantation is still an unproven treatment and while there are certainly cases of reported recovery, there are also many incidences of increasing disability. Many of the clinics use the same types of stem cells to treat a host of different disorders despite the lack of evidence to support their use. The treatments are costly and the risk to patients is enormous. If you are interested in stem cell therapies for yourself or a loved one, it is strongly advised to go through reputable routes and access registered clinical trials.
Risks and Limitations of Stem Cell Therapy
It must be cautioned that stem cell therapy is still an experimental technique that is not ready to be adopted as the standard of care for patients with CP. As mentioned previously, the risk of tumour formation is a hurdle that must be overcome. This is an important issue to address, because once injected into the body, stem cells cannot be removed. At present it is still not well known as to whether or not the “reprogramming process” of ESCs and iPSCs to more differentiated cell types is a leading cause of tumour formation. Several research projects are currently underway to reduce and if possible eliminate the generation of rogue stem cells that could be tumour-forming. The issues of “scale-up” and creating well-defined regulatory guidelines for the use of stem cells in the clinic must also be addressed.
The promise of stem cell therapy is great and heralds a potential revolution in medicine by providing new therapies for previously untreatable conditions. However, it is unlikely to provide a one-stop magic bullet to alleviate all clinical symptoms. In the case of CP, stem cell therapy is likely to result in small incremental improvements in function that in turn will lead to noticeable improvements in the quality of life for most patients. Additionally, at present it is not known for how long the beneficial effects of stem cell transplantation will last, and if multiple transplantations of stem cells over several years will be required to improve or maintain functional recovery.
As a community, it is important that we manage our expectations of stem cell therapies. As mentioned above, this treatment is still undergoing development and is not yet widely accepted in the clinic. While stem cell therapies have huge potential for the future treatment of CP, the study of these cells is a lengthy process that requires extensive investment of time and money. Support of the research that is moving stem cell therapies forward will help to fasttrack this therapeutic to the forefront of medicine within the next 20 years.
Next Steps
Now that you have a basic understanding of the state of stem cell therapies in the laboratory and clinic, we would like to provide you with some reputable sources to help you learn more. For more details on stem cell therapies, please see the following links:
Basics of stem cells:
Stem cells in CP:
Information on clinical trails:
Stem cell tourism:
Table 1: The Pros and Cons of Different Stem Cell Types
Glossary
Perinatal – Around the time of birth
Central nervous system — The brain and spinal cord
Neurons – Cells in the brain that send signals in order to communicate with other regions of the brain and the body.
Axons – Long processes attached to neurons that relay electrical signals. Analogous to wires in an electrical circuit.
Oligodendrocytes – Produce myelin, a fat that insulates neuronal axons so that neurons can send signals quickly.
White matter tract – A bundle of axons connecting two different regions of the central nervous system.
Regenerative therapy — A therapy that seeks to restore the integrity of damaged tissue. Regenerative therapies using stem cells often seek to replace dead cells and support the remaining cells.
Differentiate — The ability to transform into specialized cell types found in the body. Once differentiated, the cells cannot go back to their previous state.
Pluripotent – A term used to describe certain stem cells that can differentiate into all the different cell types found in the human body.
Somatic cell – A fully differentiated cell found in the human body (for example, a skin cell).
Directly reprogrammed cell – A somatic cell that has been directly transformed into the desired cell type. This cell has by-passed the pluripotent state that is required when making iPSCderived cells (see section on induced pluripotent stem cells).
Multipotent — Stem cells that can only differentiate into specific cell types. For example, a neural precursor cell can only differentiate into the cell types found in the brain and spinal cord and not into cell types found in the skin.
Allogeneic stem cell transplantation – Stem cells that are taken from a donor and given to a patient. Poses a high risk of immune rejection.
Autologous stem cell transplantation – Stem cells that are taken from a patient and after culturing, are transplanted back into the patient. Minimal risk of immune rejection, but the preparation of the cells takes a long time.
Endogenous – Residing within a tissue. This is opposed to “exogenous”, which means from “outside” the tissue.
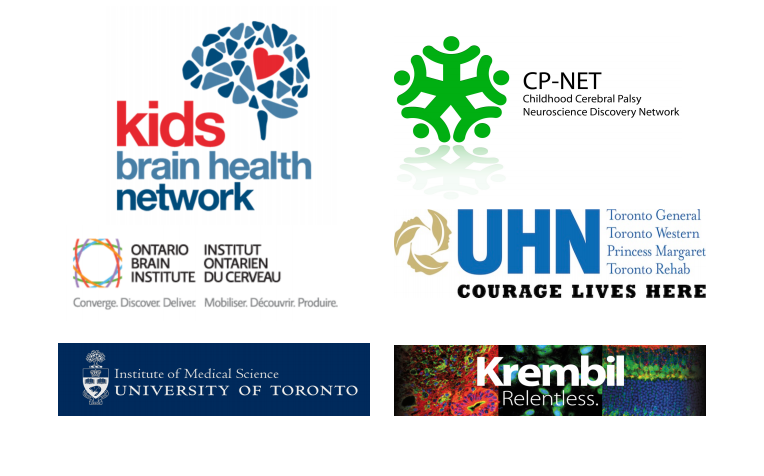